Understanding the mechanisms of adaptation to climate change: a real challenge
Growing urbanization, intensive agriculture, overexploitation of resources, introduction of exotic species and climate disruption: all these environmental changes make for an explosive cocktail for biodiversity.
Céline Teplitsky, University of Montpellier; Anne Charmantier, Center national de la recherche scientifique (CNRS) and Suzanne Bonamour, Sorbonne University
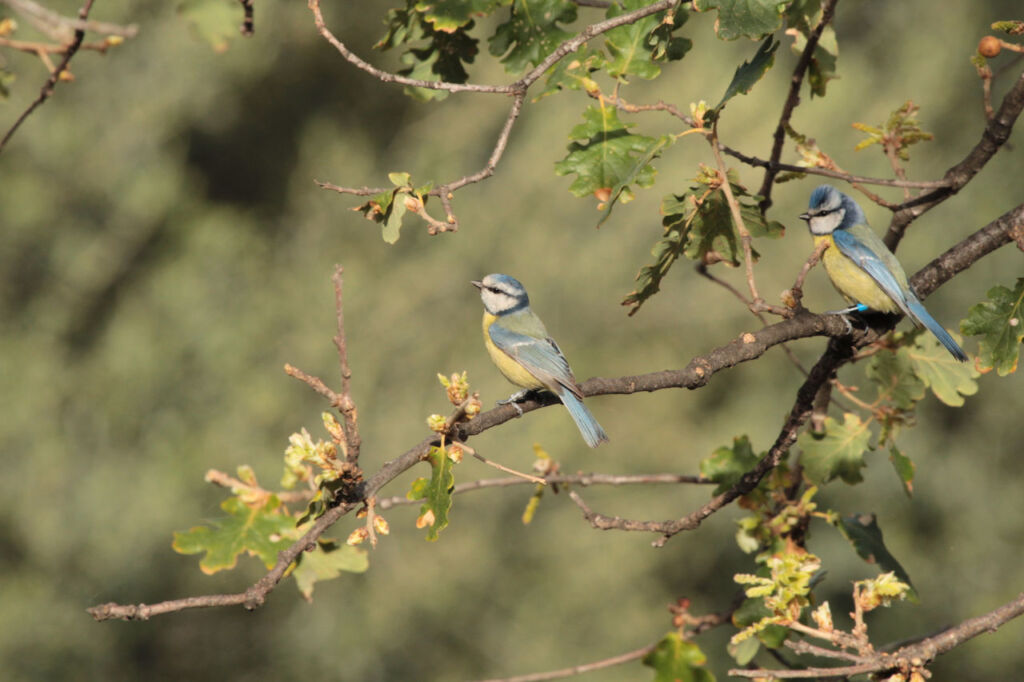
Faced with these transformations, questions frequently arise: will wild species be able to adapt to such rapid and far-reaching changes? Will the adaptations observed enable us to preserve part of the world's biodiversity?
Over the last few decades, our understanding of how living organisms adapt to their environment has changed dramatically.
For a long time, it was thought that species evolved over very long timescales, before it was realized that evolution could be very rapid, as demonstrated by the evolution of antibiotic resistance in bacteria that are pathogenic to humans, or the evolution of coloring in a common butterfly, the job moth, following the blackening of tree bark due to heavy air pollution in 19th-century England.
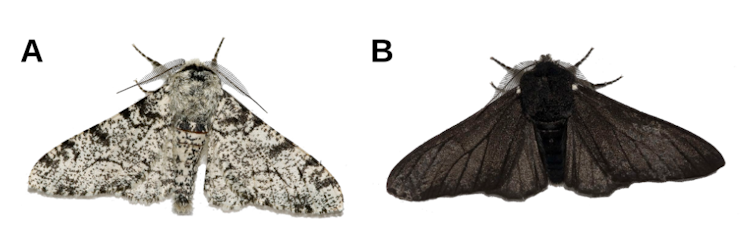
Vincent Guili, CC BY-NC-SA
Evolution and plasticity
Living organisms adapt to changes in their environment (such as reduced rainfall or the presence of a new predator) via two major processes: genetic evolution and/or phenotypic plasticity.
Adaptation through genetic evolution is achieved by modifying the genetic composition of the population between generations, through the action of natural selection. For example, mosquitoes carrying a new mutation that appeared in the 1980s survive much better against insecticides than other individuals not carrying this genetic innovation. As a result, this mutation and the insecticide resistance it provides have spread throughout natural mosquito populations in around two decades.
This process of adaptation by evolution of genetic composition requires that the traits selected (resistance, for example) are at least partly "heritable" - i.e. transmissible between generations, from parents to children - and genetically variable. It's important to note that since genetic changes take place between generations, the shorter the generation time of the species, the faster the genetic evolution: for example, the mosquito can adapt to a new environment faster than the whale.
A second adaptation mechanism is "phenotypic plasticity".
Whereas genetic evolution is a process that induces changes between generations in a given population, phenotypic plasticity is an adaptive process that can induce changes within each individual of the population.
For example, in many mammals, the amount of adipose tissue in an individual can vary according to a number of environmental parameters, including cold. Similarly, many species increase their vigilance time when the risk of predation is high.
Plasticity is thus expressed within a generation, enabling faster adaptations than through genetic evolution. In particular, it enables the organism to readjust in response to changing environmental conditions over the course of an individual's life.
Often, organisms need time to get ready for new environmental conditions: if the plastic response is the development of a defense against predators, this must be put in place well before the encounter with the predator. Organisms therefore use cues present in the environment to develop the right response at the right time. This is the case with tadpoles, which develop different morphologies depending on the presence or absence of predator scent.
In the current context, the existence of a mechanism such as phenotypic plasticity, which is widespread in the living world and can enable rapid adaptation to environmental changes, is of major interest in understanding and anticipating the consequences of anthropogenic upheavals on biodiversity.
Pondering at the right time
The blue tit(Cyanistes caeruleus), a small passerine bird, is much studied in the discipline of ecology, and is the subject of much research based on observations in natural populations.
Work on this species has contributed to a better understanding of the importance of phenotypic plasticity in the adaptation of organisms to climate change.
Studies carried out in the wild over the last few decades have enabled us to gain a better understanding of the ecology of the blue tit. In this species, as in most other insectivorous passerines living in temperate forests, chicks are mainly fed caterpillars by their two parents - around 1,800 caterpillars to feed a single chick from hatching to fledging.
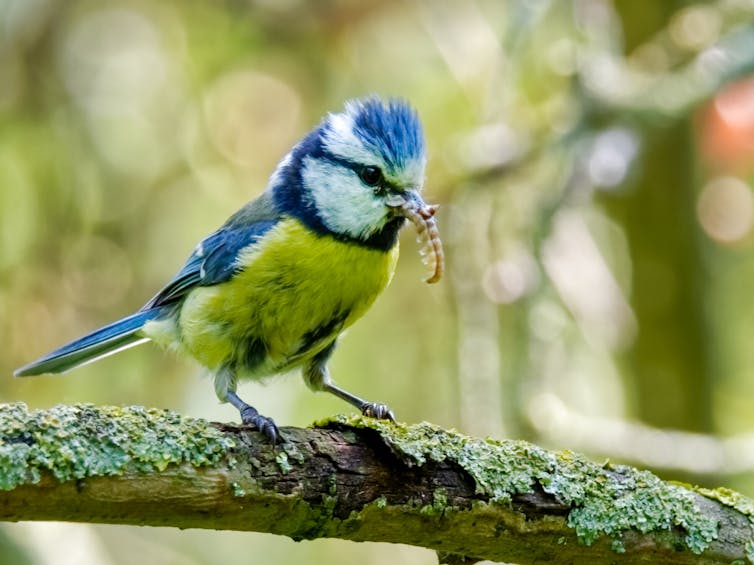
Lumiks Lumiks/Flickr, CC BY-NC-SA
Synchronization between the chicks' need for food and the period of caterpillar abundance has a major impact on chick survival. To ensure that the chicks are born at the right time, i.e. when the parents can find a large quantity of caterpillars to bring back to the nest, the female chickadee must lay eggs around 30 days before the peak of caterpillar abundance in the forest.
But how do you lay eggs at the right time?
The date on which tits lay their eggs, as with many birds, depends in part on the environment, and in particular temperature: in warm years, tits lay their eggs earlier than in cold years, perfectly illustrating the concept of phenotypic plasticity.
The period during which chickadees are most sensitive to temperature, i.e. the period when birds pick up on signs of a warmer or earlier spring, can vary from one to three months before breeding. Depending on the population, this period of temperature sensitivity begins in spring or late winter. The reliability of temperature as a predictor of the period of food abundance (caterpillars) is crucial to breeding success.
Plasticity in response to temperature is common. In response to global warming, many plant and animal species are reproducing earlier and earlier, just as trees are budding earlier. These changes in life rhythms, linked to the response of organisms to temperature changes, can already be observed in our gardens and forests.
Plasticity therefore goes a long way towards explaining what we call increasingly early springs. Phenological plasticity - i.e. the timing of events throughout the year, such as the laying date of chickadees - is one of the main responses of wild species to climate change.
However, little is known about how global change may affect this response and test the limits of adaptation. Is it possible for species to adapt rapidly to these novel and stressful environments? A recent study suggests that phenological plasticity may already be insufficient to allow populations to persist.
Also read:
Climate change and the biodiversity crisis: a dangerous alliance
New and stressful disruptions
On the question of animal adaptation to climate change, many scientific questions remain unanswered.
What happens when environments become too different from those historically experienced by organisms? In particular, how does adaptation to gradual climate change enable us to cope with extreme climatic events such as heatwaves? How will differences in responses between species affect their interactions (e.g. between prey and predators, or between cooperating species)?
Caterpillars are developing earlier and earlier in response to climate change, but is there a limit for chickadees, a date before which it is physiologically impossible to start breeding, preventing synchronization with their prey? How do global changes affect the reliability of information needed by organisms to respond to the environment?
For example, when they hatch, sea turtle hatchlings often head for the city rather than the sea, as urban light is stronger than lunar light. Could this type of misinterpretation limit or even cancel out the benefits of plasticity?
Finally, will organisms be able to cope with multiple environmental changes thanks to phenotypic plasticity? In addition to climate change (changes in temperature and precipitation), organisms are confronted with multiple disturbances - new pathogens and predators, the presence of pesticides, the development of urban environments, etc. - that can affect their ability to adapt to these changes.
Our "Mommy knows best" research project aims to assess whether the limits of plasticity are already detectable in natural populations, using the blue tit as a study model. The aim is to test the effect of various environmental factors on the plasticity of laying date in chickadees (such as the effect of urbanization or agricultural practices, for example) and to model the effects of plasticity on chickadee population dynamics, in order to understand to what extent changes in plasticity may affect the risk of population extinction.
The findings of this project could also shed conceptual light on the process of phenotypic plasticity in the context of climate change, and be applied to other wild species.
Understanding the limits of adaptation in the face of global change will help us to better understand the scale of the challenge facing biodiversity today... But there's no need to wait to fight against its annihilation, which is already underway!
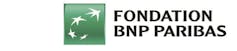
The "Mommy Knows Best" research project, of which this publication is a part, was supported by the BNP Paribas Foundation as part of the Climate and Biodiversity Initiative..
Céline Teplitsky, Researcher in evolutionary ecology, CNRS, University of MontpellierAnne Charmantier, Director of research in evolutionary ecology, Center national de la recherche scientifique (CNRS) and Suzanne Bonamour, Post-doctoral researcher, Muséum national d'histoire naturelle, Sorbonne University
This article is republished from The Conversation under a Creative Commons license. Read theoriginal article.