New structures identified in our chromosomes
Since the beginning of this century, major technological advances at the molecular and genomic level, coupled with new approaches in very high-resolution photonic microscopy, have revealed new chromosome structures and new principles of genome organization (the genome contains all genetic information and therefore all DNA sequences transmitted through cell divisions).
Frédéric Bantignies, University of Montpellier and Giacomo Cavalli, University of Montpellier

This knowledge is crucial to our understanding of how our cells function, but also to a better understanding of certain diseases and their evolution.
Deoxyribonucleic acid, or DNA, contains the genetic information specific to each living species. Chromosomes are the physical carriers of this information. The set of chromosomes is also called the genome, and contains all the genes. Since the discovery of DNA's double-helix structure in 1953, the question of how it is organized within the nuclei of our cells has been the focus of much research worldwide. To get a clearer idea of the issue, let's reiterate that human beings have 46 chromosomes, containing 3 billion base pairs (bp) of DNA - a molecular filament some 2 m long. This filament is capable of being contained in cell nuclei around 10 μm (one micrometer = one thousandth of a millimeter) in diameter. To better understand how the genome and our genes work, it was therefore essential to know how all this DNA is able to fold up in a cell nucleus.
At the beginning of this organization is the nucleosome, whose structure was elucidated in 1997. The nucleosome is made up of very basic proteins called histones, which have a high affinity for the acidic DNA molecule. These histones form a central body around which the DNA molecule wraps, at a rate of 146 bp per nucleosome, a sort of "macaroon" 11 nm (one nanometre = one millionth of a millimetre) wide and 6 nm high. The nucleosome thus represents the first structural level of DNA organization in nuclei. A sign of its importance, this very particular structure is found in all organisms with nuclei (eukaryotes), whether unicellular or more complex, such as animals and plants.
For a long time, it was thought that the succession of nucleosomes (also known as chromatin fibers), forming a sort of "string of pearls", in turn wound regularly to form a fiber 30 nm in diameter. Spiral supercoils of this fiber would eventually lead to chromosomes. However, technical limitations, including the low resolution of microscopy techniques and the absence of other methods, meant that this hypothesis could not be proven correct.
On the other hand, it has been known since 1985 that the organization of chromosomes in cell nuclei is not random. What's more, the well-known X-shape of chromosomes is not false, but represents only a very transitory stage in their organization. Indeed, this highly condensed X conformation is conducive to their segregation (sharing) in daughter cells during cell division. For the rest of the time, however, the shape of the chromosomes is quite different. Visualization of chromosomes using fluorescent molecules capable of interlocking specifically with the DNA double helix has shown that each chromosome occupies its own territory within the nucleus, avoiding excessive entanglement with other chromosomes. This "chromosome territory" property is also found in most species and seems to be very important, especially for species with a large number of chromosomes.
New technologies bring new insights into genome organization
At the beginning of the 21st century, research around the world led to a better understanding of the different levels of genome structural organization between the nucleosome and the chromosome territories.
These advances were made possible by the development and use of entirely new technologies. First came new genomics techniques, including ultra-high-throughput DNA sequencing (next-generation sequencing) and the ability to molecularly capture fine chromosome structures using the "Hi-C" method. At the same time, the arrival of super-resolution photonic microscopy, using fluorescent DNA markers, has made it possible to visualize these chromosome structures directly in the cell nucleus.
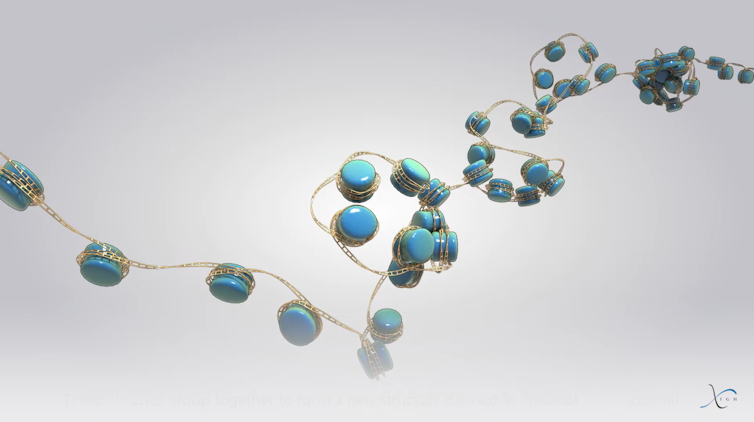
Frédéric Bantignies, Giacomo Cavalli, Provided by the author
So let's return to our chromosome organization ladder. Its first level is the nucleosome. A second level of organization corresponds to groupings of a few nucleosomes, like little clusters called "nucleosome clutches" (so named by their discoverers in analogy with the eggs found in incubated nests). Nucleosomes are therefore not grouped in a regular fashion as previously thought, but rather in irregular clusters.
These "nucleosome clutches" then group together to form a structure called a "Chromatin Nanodomain", or CND, which includes approximately 100,000 to 200,000 base pairs of DNA, forming large irregular clusters of nucleosomes 150 to 300 nm wide. These two levels were discovered recently (in 2015 and 2020 respectively), thanks to super-resolution microscopy, capable of resolving structures from 20 to 100 nm.
TADs structure the genome and regulate gene expression
The next level of this organization is called TADs (Topologically Associating Domain), identified in 2012 using the Hi-C molecular method. TADs are composed of several CNDs, forming superclusters of nucleosomes around 500 nm wide. They thus comprise variable sizes of DNA, with an average of around 1 megabase (1 million base pairs). Our laboratory contributed to the discovery of CNDs and TADs.
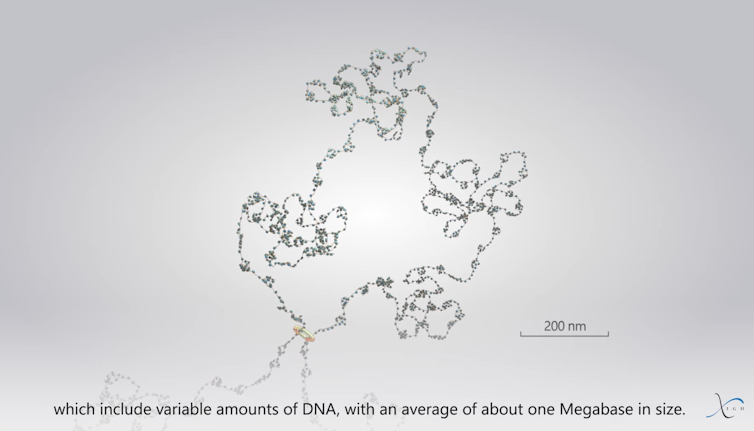
Frédéric Bantignies, Giacomo Cavalli, Provided by the author
TADs are quite heterogeneous structures, not least because of their dynamic formation mechanism. This mechanism involves the passage of the famous "string of pearls" (referring to the succession of nucleosomes) through the ring formed by cohesin. The chromatin fiber continues to pass through the ring until, at the borders of a TAD, it encounters nuclear factors called CTCFs, a kind of "customs officer" which, positioned on the DNA, blocks the fiber's progress. As the fiber passes through, the nucleosomes organize themselves into clutches and CNDs. The TAD then represents the whole of this large chromatin loop that has passed through the cohesin ring.
Within chromosomes, gene activity is influenced by a whole host of regulatory sequences (switches of sorts) that can be tens of thousands of base pairs away from their gene. Thus, TADs will preserve genes and their regulatory regions in the same molecular environment, which may be conducive to their expression (i.e. their reading to lead to the production of a protein) in a given cell type where their activity is required. Thanks to their borders, they will also enable genes to be separated from each other, to avoid active genes influencing other inactive genes in a given cell type.
Recent studies have shown that chromosomal defects at the boundaries of TADs (such as inversion or deletion affecting CTCF positioning or rendering it inoperative) lead to defects in isolation between genes, and hence to erroneous gene activation. In some cases, these rearrangements lead to the activation of genes known as "proto-oncogenes", which can lead to cell transformation and the appearance of tumors.
Genome compartmentalization and territorialization
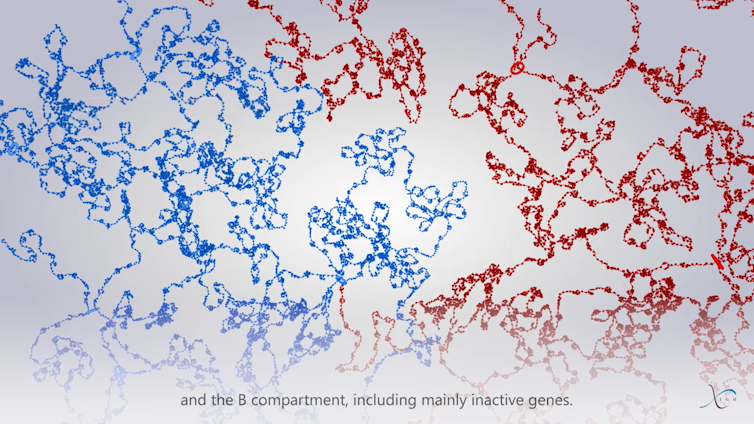
Frédéric Bantignies, Giacomo Cavalli, Provided by the author
Several TADs are then grouped into two distinct compartments: compartment "A", which contains mainly active genes, and compartment "B", which includes mainly inactive genes. This grouping into compartments enhances genome functions.
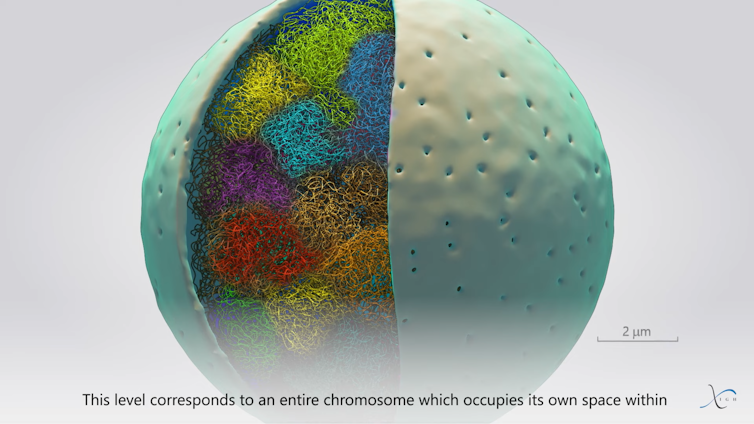
Frédéric Bantignies, Giacomo Cavalli, Provided by the author
Finally, at the end of this organizational scale, we find the chromosome territories, which allow chromosomes to be individualized from one another within the nuclei. This organization plays an important role in DNA replication (identical copies of genetic material) and cell division, where each duplicated chromosome is split in two in the daughter cells.
And we've come full circle! Well, almost: we now need to better understand how this organization influences the various molecular processes inherent in the genome, which regulate gene expression, DNA molecule replication, repair in the event of damage or stress, and recombination, particularly in reproductive cells. The field of investigation remains immense, but a better understanding of genome organization will pave the way to a better understanding of all these nuclear processes in normal cells, and of course also in cells with chromosomal defects that lead to diseases such as cancer.
Frédéric Bantignies, CNRS Research Director, University of Montpellier and Giacomo Cavalli, CNRS Research Director, University of Montpellier
This article is republished from The Conversation under a Creative Commons license. Read theoriginal article.