What if the Y chromosome hadn't evolved as expected?
It's one of the best-known facts of genetics: women are XX and men XY. In humans, chromosomes determine sex, and this particular pair, the 23rd, is the most astonishing. It's the only pair of chromosomes where two versions with enormous differences coexist. So much so, in fact, that it's difficult to recognize two chromosomes in this pair that were ancestrally very close, but which, over millions of years of evolution, have become unrecognizable.
Thomas Lenormand, University of Montpellier and Denis Roze, Sorbonne University
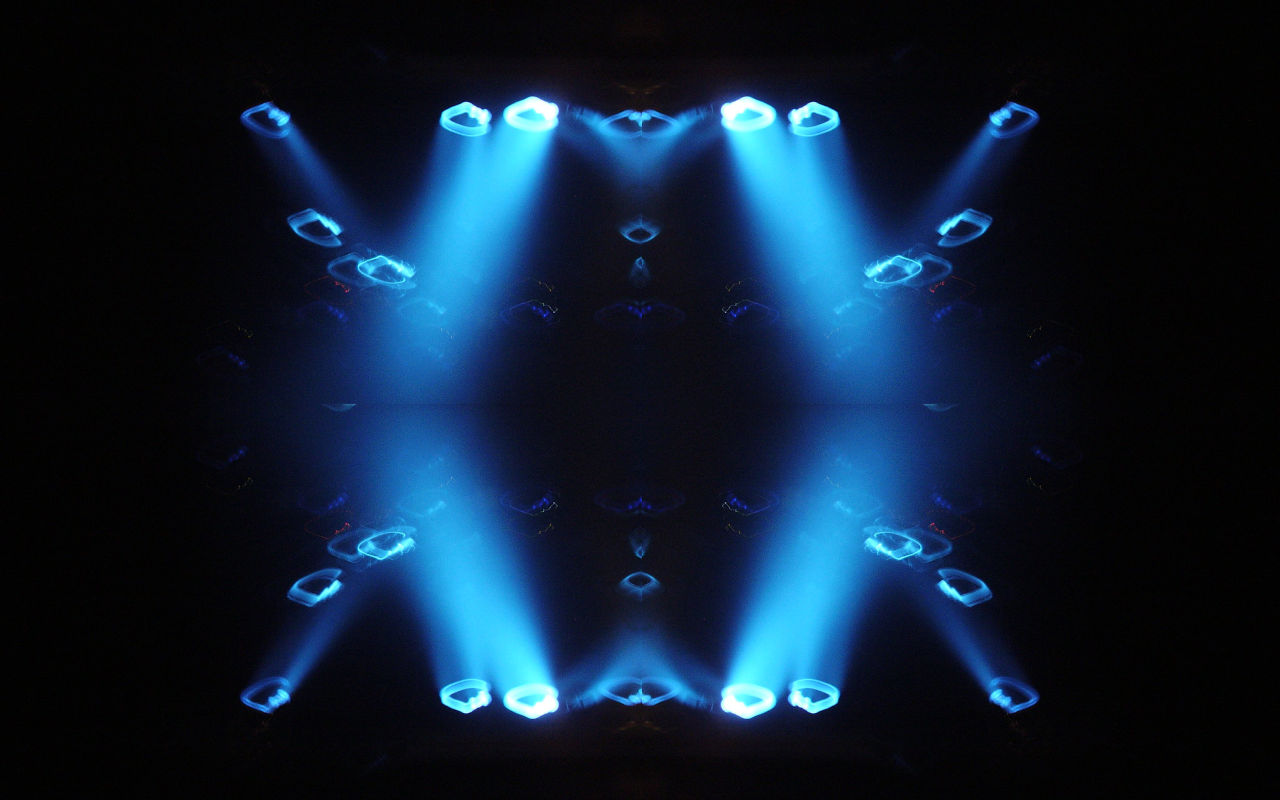
Why did sex chromosomes evolve to become so unique? This question has been nagging geneticists for almost a century. Several hypotheses have been put forward over the years, and a theory has gradually been consolidated. However, this long theoretical construction has just been turned upside down by a new model that calls this edifice into question.
The X-Y dichotomy
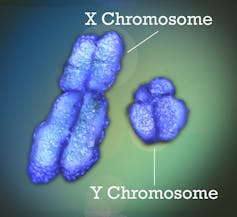
NIH/Flickr, CC BY-NC
The X is a fairly ordinary chromosome, with almost 800 protein-coding genes. The Y, on the other hand, is much stranger: smaller (about a third the length of the X), it has only about 60 genes left. However, these two chromosomes do form a "pair". During sperm production, they pair up and recombine on a small portion (recombination is an exchange of genetic material, in this case between the two chromosomes of the same pair), testifying to their ancestral homology. But 95% of Y no longer recombines at all. X, on the other hand, recombines along its entire length in XX females during egg production.
Finally, another oddity: only one X is expressed in females. In other words, genes are read to produce proteins from a single X, either the one inherited from the father, or the one inherited from the mother, randomly depending on the cell. This enables "dosage compensation", so that the genes carried on the X are expressed at the same level in males and females.
Widespread differences in living organisms
One might think that these eccentricities are unique to humans. In fact, they're extremely common in animals and even plants, although the details vary.
However, on a very regular basis, recombination is stopped on the sex chromosomes, a non-recombining chromosome (Y) with few functional genes (it is "degenerated"), and dosage compensation.
These regularities soon caught the eye of geneticists, who began looking for a theory to explain why recombination stops on these chromosomes, and why this stop in recombination is associated with degeneration. These same geneticists also quickly realized that answering this question might also shed light on a general question that was bothering them even more: the role of recombination in evolution.
The role of recombination
Recombination, when gametes are produced, enables genetic "mixing". This is an essential feature of sexual reproduction, and undoubtedly the key factor explaining the advantage of sex over clonal asexual reproduction. The degeneration of non-recombining sex chromosomes seems to be life-size proof of the importance of recombination in maintaining genome integrity, in the face of the constant flow of deleterious mutations altering genetic information.
Indeed, most chromosomes carry mutations, which can be passed on from one generation to the next if their effect on organism survival or fertility is not too great. The mutation-free version of a chromosome fragment can be quite rare in a population, and may even disappear if the individuals carrying it leave no descendants. When this happens, a mutation-free fragment can only be recreated by recombination between chromosomes carrying mutations in different places. As a result of this process, non-recombining areas of chromosomes are expected to gradually accumulate mutations.
The absence of recombination could therefore explain Y degeneration. But this doesn't entirely resolve the question: why on earth do sex chromosomes stop recombining if this stop leads to degeneration?
The sex-antagonist gene theory
Until our work, this was explained by the theory of "sex-antagonistic" genes. Males and females differ in many characteristics: this is sexual dimorphism. For many genes, there are versions ("alleles") that are advantageous for one sex and disadvantageous for the other.
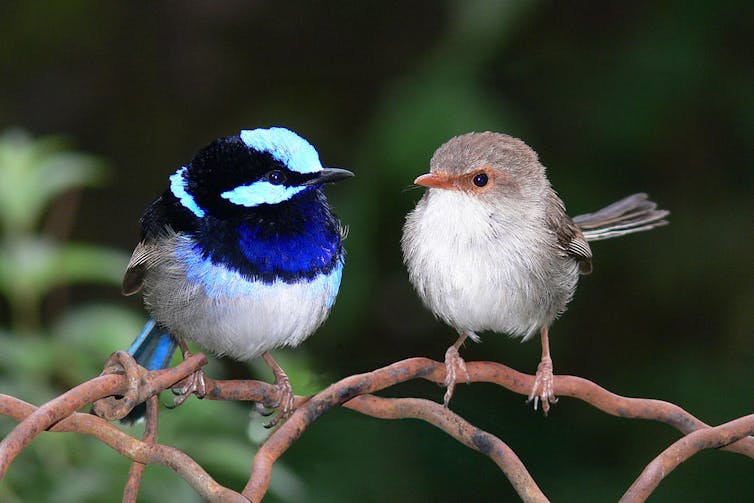
benjamint444/Wikimedia, CC BY-SA
If these "sex-antagonistic" genes are frequent, we can expect to find them on the sex chromosomes. Suppressing recombination (and therefore the exchange of alleles between the X and Y chromosomes) can then become advantageous. If an allele that is advantageous in males is found on a Y that no longer recombines, it will remain on that Y: it will always be found in males, but never in females.
A global theory of sex chromosome evolution in three stages has thus emerged. Stage 1 is the cessation of recombination on the Y, caused by the presence of sex-antagonistic genes on the sex chromosomes. Stage 2 is the accumulation of deleterious mutations (degeneration), following recombination arrest. Stage 3 is the evolution of dosage compensation to compensate for the lack of expression of X genes in males.
This theory had the advantage of being very elegant, because it was very general. However, a number of subsequent observations have gradually called this edifice into question, and reopened the theoretical debate.
A new theory
To do this, we started with the only "ingredients" that seem essential to explain the evolution of sex chromosomes: recombination arrest events on the Y (but which are not irreversible), deleterious mutations (degeneration cannot take place without them), and regulators of gene expression that can modulate their expression levels (dosage compensation cannot take place without them).
To our great surprise, these ingredients - and in particular the last one that had never been formally incorporated into classical theory - proved sufficient. Our research uncovered an overall process entirely different from the "classical" theory, in which all the causal relationships are reversed, and the "sex-antagonistic" genes become unnecessary.
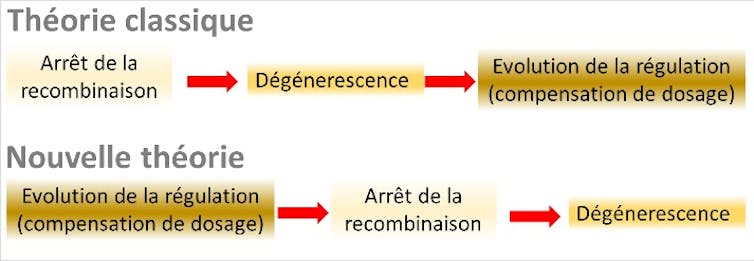
Thomas Lenormand, Provided by the author
Like the classical theory, this new theory assumes an ancestral situation in which a gene carried by a pair of "normal" chromosomes (autosomes) determines the sex of individuals. Two different forms of this gene (or alleles, denoted M and F) coexist, with MF individuals developing into males and FF individuals into females.
It is then assumed that chromosomal inversions can occur: an inversion corresponds to a "flipping" of a part of the chromosome, with the genes located in this part being found in a reversed order. Inversions are a relatively rare form of mutation, but nevertheless occur from time to time in populations. Their effect is to prevent (at the level of inversion) recombination between inverted and non-inverted chromosomes. This is because the different order of the genes prevents the chromosomes in this zone from pairing up correctly.
When an inversion encompassing the M allele (determining male sex) occurs, it may have an advantage if the genes it contains carry fewer deleterious mutations than average. In this case, it will increase in frequency over the generations, until all males carry this inversion. As a result, chromosomes carrying F and M alleles become X and Y "proto-chromosomes", no longer recombining along part of their length (in the inversion zone).
However, the cessation of recombination eventually causes an accumulation of deleterious mutations on the chromosome carrying the M allele (the chromosome carrying the F allele continues to recombine in females). This accumulation of mutations can lead either to a return to recombination (for example, by a new chromosome inversion restoring the original gene order), or to a reduction in the expression of mutated genes carried by the proto-Y, which will be compensated for by an increase in the expression of proto-X genes: this is the start of dosage compensation. When this process is set in motion quickly enough, it prevents any backtracking: a restoration of recombination will destroy this delicate balance between the expression levels of the X and Y genes.
The computer simulations we have carried out show a gradual halt to recombination, in successive "strata" corresponding to different inversions stabilized by the evolution of dosage compensation, with no need to invoke the existence of sex-antagonistic genes.
By completely renewing our vision of possible scenarios for the evolution of sex chromosomes, this new theory opens up a vast field of experimentation and empirical testing. It also shows the importance of taking into account the regulatory mechanisms of gene expression in evolutionary theory. Finally, it could suggest that taking into account the evolution of regulators could be one of the keys to solving the mystery of maintaining sexual reproduction in plants and animals.
Thomas Lenormand, Research director, University of Montpellier and Denis Roze, Researcher in evolutionary genetics, Sorbonne University
This article is republished from The Conversation under a Creative Commons license. Read theoriginal article.