We inherit our parents' genetics, but what about epigenetics?
Epigenetic marks are chemical modifications of DNA that activate or switch off certain genes. They are reproduced when cells divide, but can they be reproduced between two generations?
Bantignies Frédéric, University of Montpellier and Giacomo Cavalli, University of Montpellier
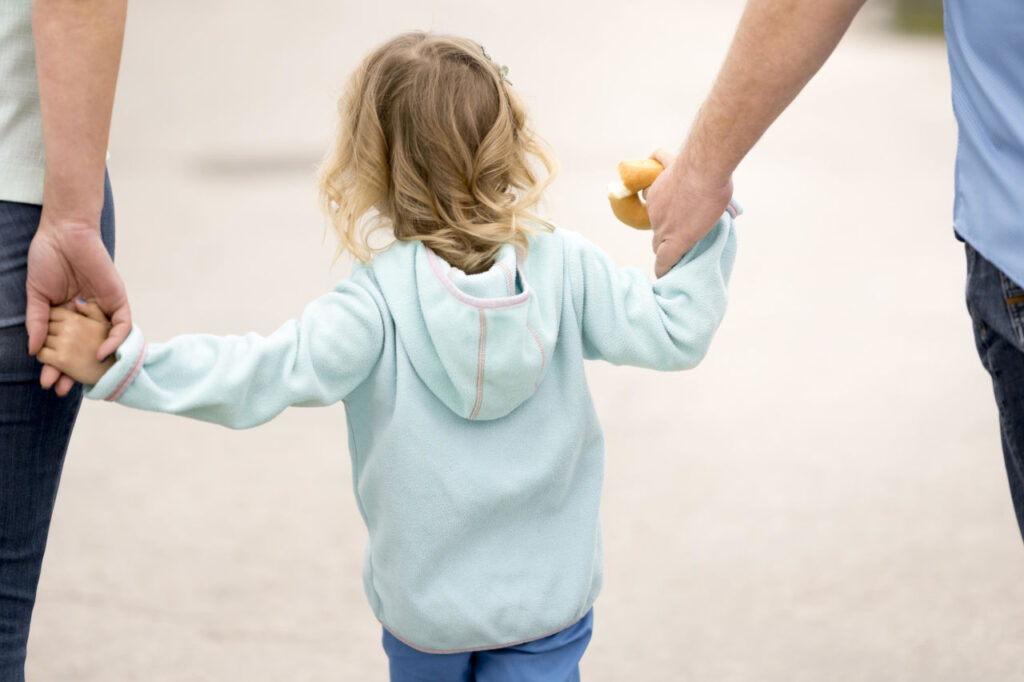
At the base of genetic information, DNA is the founding molecule of life on earth. However, it cannot function alone, as its information must be interpreted by proteins present in the cell nucleus, adding a further layer of information known as epigenetic information ("epi" coming from the Greek prefix meaning "above").
In each generation, we inherit the DNA contained in the chromosomes of both our parents, in the form of 23 chromosomes from the mother and 23 chromosomes from the father, culminating in the 46 chromosomes of the human species (this number varies according to species) in each of our cells. During development, our cells divide a very large number of times to form adult individuals (made up of over 30,000 billion cells!).
The equation seems relatively straightforward, since the DNA sequence of the 46 chromosomes is copied identically (during "DNA replication") before being divided equally into two "daughter" cells during each cell division (called "mitosis"). Despite this identical genetic information, our cells are not all the same. Each of them differentiates to produce the variety of tissues and organs that make up our body. It's epigenetic information that makes this differentiation possible.
Bookmarks in our genome
What do we know about these epigenetic mechanisms, which have been the subject of intense research since the turn of the century? They involve a large number of cellular factors, the function of which we are beginning to understand. Within chromosomes, negatively-charged DNA is wrapped around positively-charged proteins known as histones. Hundreds of factors bind, either directly to DNA or to histones, to form what we might call an "epigenetic sheath".
This sheath is far from homogeneous. It contains factors that can deposit small molecules on the DNA molecule or histones, acting like "bookmarks" that could be placed in a book at precise points. These are the famous "epigenetic marks" (methylations, acetylations...) and a large number of them are found at the level of genes located all along our chromosomes. These marks are indispensable, differing in the different cell types that make up an organism, and contribute directly to the regulation of gene expression (for example, genes important for liver function will be expressed in liver cells, while genes specific to neurons or muscle cells will be switched off there). The question now is whether these epigenetic mechanisms also influence the transmission of genetic information.
From Monday to Friday + Sundays, get free analysis and decryption from our experts, for a different take on the news. Subscribe today!
Let's start with the simplest case of mitosis (cell division). Most cells must pass on their function, i.e. reproduce the expression of their specific set of genes in their daughter cells. A major upheaval occurs during mitosis, when chromosomes, and therefore the DNA making them up, are grouped and condensed. During this stage, DNA remains wrapped around histones, but many sheath proteins are dissociated. On the other hand, most of the marks on the DNA and histones are retained. Epigenetic markers will thus remain on and around the DNA. Once mitosis is complete, these marks will enable the daughter cells to rapidly reconstruct the architecture of the genes along the chromosomes, which will still bear the essential epigenetic marks and will once again be able to recruit the factors associated with their function. Epigenetic mechanisms are thus directly involved in the inheritance of cellular function.
Could this epigenetic information also be passed on to future generations? In this case, the challenge is much greater. The information must be transmitted to the male and female gametes, which fuse during fertilization to form the first cell of the embryo. This cell will divide to form the different cell types of the future organism. Chromosome reorganization and condensation are even more drastic than in mitosis. DNA methylation, histone marks and the 3D organization of chromosomes are largely reprogrammed, as if the organism were striving to erase the epigenetic baggage accumulated in the previous generation.
Epigenetic transmission in certain species
Can epigenetics nevertheless influence the genetic inheritance of offspring? Today, this question is the subject of intensive, and sometimes controversial, research. In particular, this research aims to understand whether environmental, nutritional, stress-related or chemical/physical exposure factors could have an influence on future generations.
Precise examples of the effect of the environment on epigenetic regulation have been described in numerous animal and plant species.
In reptiles, temperature is a major determinant of sexual type, via the deposition of specific epigenetic marks (histone methylation). In insects, many phenotypic traits are linked to diets that lead to epigenetic variations, such as the distinction between queen and worker bees, or the distinction of worker castes in certain ants (via DNA methylation and histone acetylation, respectively).
Exposure to chemical factors such as arsenic or bisphenol A can also cause epigenetic modifications. We are currently trying to understand the mechanisms by which these stimuli act, and whether they can generate a stable inheritance over generations in the human species. Epidemiological studies have attempted to establish links between diet and influence on offspring, but these studies often come up against multi-factorial aspects that are difficult to control, and suffer from a lack of molecular evidence.
Studies at the level of model organisms are therefore needed to investigate the influence of epigenetic modifications on the transmission of genetic information. Examples of the transmission of epigenetic states across generations are currently well described in the worm C.elegans and in plants, organisms where there is less reprogramming of the epigenetic state at fertilization. Our team is working on the model organism Drosophila melanogaster or vinegar fly. Following disruptions to the 3D organization of chromosomes, we have been able to demonstrate the inheritance of epigenetic modifications to a gene responsible for eye color over many generations.
In the mouse model, studies have also shown that the insertion of genetic elements can induce epigenetic modifications, notably DNA methylation. These modifications lead to the repression of a gene responsible for coat color, or of important metabolic genes which, when deleted, cause obesity or hypercholesterolemia, in a way that can be inherited over several generations.
In these examples of transgenerational inheritance, the first signal to induce an epigenetic change involves the modification of a small DNA sequence. Even if this sequence is restored to normal, the change is maintained. Although probably rare and possibly influenced by genetic inheritance, these phenomena could exist in nature. Future research will be needed to understand whether other epigenetic mechanisms could be inherited.
Although these data do not change current genetic laws, they do indicate that, beyond the simple duplication and transmission of the DNA sequence to male and female gametes, epigenetic information could contribute to the inheritance of certain traits in many species. This research could therefore help us to better understand the evolution and adaptation of species, as well as the mechanisms underlying certain human pathologies, such as cancer.
Bantignies Frédéric, CNRS Research Director, University of Montpellier and Giacomo Cavalli, CNRS Research Director, University of Montpellier
This article is republished from The Conversation under a Creative Commons license. Read theoriginal article.