Covid: a new modeling method for better epidemic risk assessment
For thousands of years, mankind has been struck by epidemics... Each time, protective measures have been taken in the context of the knowledge and even the opinions of the time on the modes of contamination. Take the plagues that ravaged Europe a few centuries ago. At the time, it was firmly believed that this devastating disease was caused by the inhalation of miasmas and the ensuing imbalance of the body's humours. Masks stuffed with medicinal herbs housed in a pointed beak flourished: the epinal image of bird-beaked masks...
Simon Mendez, University of Montpellier and Alexandre Nicolas, Claude Bernard Lyon 1 University
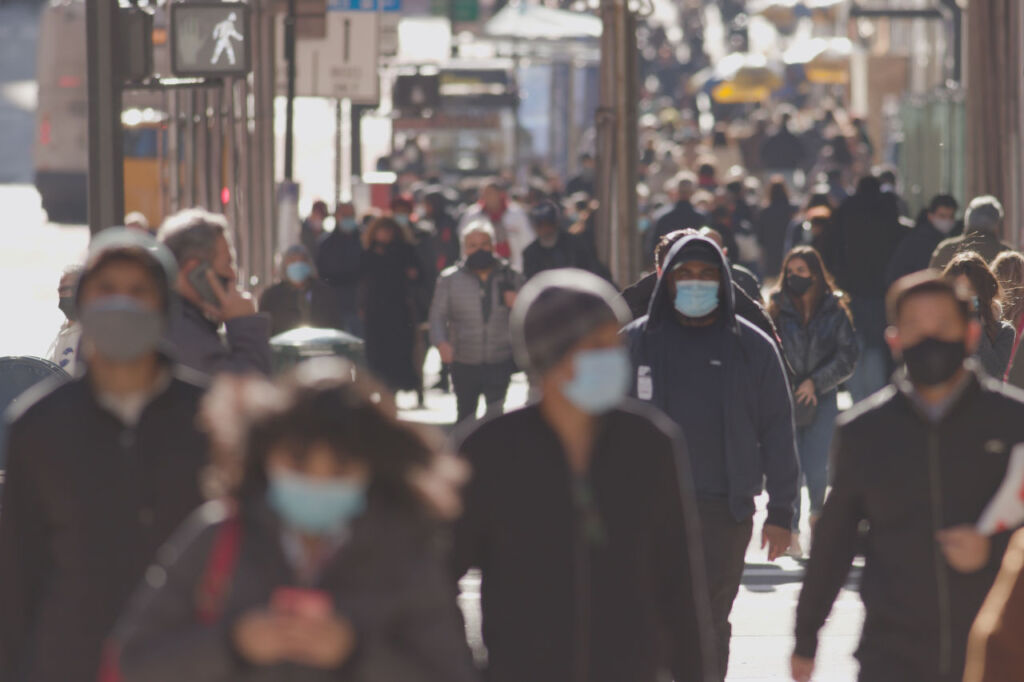
In the early 20th century, there was a major shift towards the predominant role of close contacts in transmission. Recommendations changed, and the preventive measures promoted at the start of the Covid-19 pandemic were the result of this shift: hand-washing, possibly with hydroalcoholic gel, single-use handkerchiefs, sneezing into the elbow, etc., all to ward off the risk of direct hand-carried infection or infection through the medium of an infected person. All of this to counter the risk of direct hand-carried infection, or infection via contaminated objects (known as fomites) from a contagious person.
Three years into the pandemic, the academic understanding of respiratory disease transmission has been reshuffled. What do we currently know about its mechanisms? How can we assess the risks in different situations - from a café terrace to a queue respecting a certain social distance to a busy street? We set out to answer these questions by developing a simple, rapid modeling method.
shedding light on the mechanism of aerosol transmission
At present, there is reason to believe that aerosol transmission is the dominant mechanism for the spread of Covid.
Experimental data collection on this airborne pathway began long before the pandemic: Lydia Bourouiba's group's images of the projection of micropostillons emitted during a sneeze (below), and those of Lidia Morawska and William D. Ristenpart's teams, among others, on the size of droplets and aerosols emitted during various expiratory activities, date back several years.
These studies show that coughing and sneezing project aerosols over distances potentially greater than 2 m, and that the simple act of speaking for one minute can generate as many droplets as a coughing fit.
Despite these results, disagreement persists over basic data such as the size distribution of the droplets and aerosols produced, a critical element in determining their fall time and their chance of remaining suspended in the air, in an indoor environment. What's more, experimental difficulties and ethical concerns prevent the study of all possible emission, inhalation and environmental conditions.
Digital simulation: the cure?
So what can be done to multiply testing possibilities? One answer is to use digital tools, taking advantage of the computing power of computers.
All that remains is to choose the system to be simulated: since airborne transmission takes place via the transport of viruses within droplets and aerosols formed in the respiratory tract (lined with mucus) and mouth (filled with saliva), the challenge is to simulate the propagation of these micropostillon-carriers from their emission to their inhalation - and even to their penetration and deposition in the respiratory tract.
Such fluid dynamics simulations have flourished since the early days of the pandemic, and have highlighted the complexity of the process, the importance of properly describing turbulent flow structures, the variability of the exhaled air jet according to phonation, the sensitivity of droplet evaporation to the breath environment, and so on.
If we settle for coarse models, the description of risks can be severely affected, and in the early days of the epidemic we saw numerous studies with questionable hypotheses. On the other hand, sophisticated models that simulate the propagation of droplets of respiratory fluid offer greater realism... But they are hampered by the complexity of analyzing the data produced (what can we do with the range of trajectories that vary with each microchange?) and by the cost in computing time - the lifeblood of numerical simulations.
The "dynamic risk map" solution
To get the best of both worlds, one idea is to use very fine fluid dynamics simulations, with a resolution of the order of a millimeter, and estimate the risk dynamics around an emitter in a more aggregated way, i.e. without worrying about the precise location of each micropostillion.
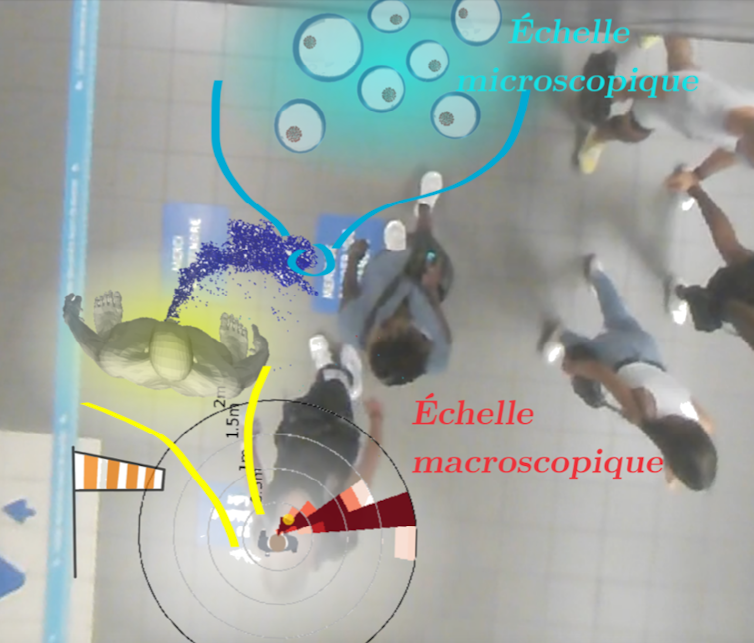
Except that a single map is not enough: in reality, the "risk map" thus obtained varies according to whether the person is talking or walking, depending on the wind or air currents, and so on. We therefore need to build up a whole library of reference situations and, in the intervals between them, infer those that are missing. That's what we've set out to do.
The results are clear and simple. For example, the slightest breeze entering the scene drastically reduces the risk of viral transmission. This brings to an end a debate that began at the start of the pandemic, when people wondered whether it might not encourage contamination by carrying droplets and aerosols further. In fact, in all the scenarios studied, it disperses them.
More generally, the significant reduction in digital costs thanks to the use of "dynamic risk maps" has made it possible to study concrete situations involving dozens or even hundreds of people.
Concrete results
In concrete terms, we were able to walk the streets of Lyon at the height of the pandemic and set up our camera (filming people from above, while respecting their anonymity) in a variety of locations, both outdoors and indoors (large, well-ventilated spaces). These included an SNCF train station, a metro station, busy streets, an open-air market, café terraces and a landscaped bank of the Rhône.
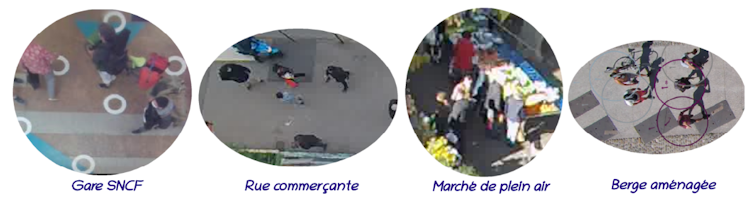
From these videos, we extracted the trajectories and orientations of the pedestrians' heads and coupled this data to the aforementioned risk maps (Figure 1). Summary :
- Passing (uncrowded) streets present a very low risk compared to the open-air market, where passers-by were much more numerous and crowded. Not surprisingly, density plays a major role.
- All these situations presented a lower risk of new contamination than café terraces (Figure 3), where people share close, prolonged contact, even though the overall density is lower.
- Expiratory activity plays a major role, since droplet emissions from a person who is talking are much higher than from a person who is breathing through the mouth (and, a fortiori, through the nose).
This prioritization of real-life scenarios based on digital-theoretical models illustrates how high-fidelity simulations can be used to examine real-life situations. This can be used as a decision-making aid in public health policy.
The modeling tool thus plays the valuable role of a bridge between fundamental knowledge of airborne viral propagation and the sanitary measures to be implemented.
Strengths and limits of a promising approach
Compared with fine approaches, where the destiny of each respiratory droplet is predicted and influenced by numerous phenomena (air recirculation around street furniture, influence of each pedestrian's wake...), ours enables us to estimate risks in real-life situations in just a few minutes, the most costly simulations having been carried out upstream, once and for all.
Of course, this comes at a price: the impact of pedestrians other than the emitter is not taken into account, which proves limiting in the case of extremely dense crowds. Other effects, however, such as the influence of temperature on the dispersion of exhaled aerosols, could be incorporated in future improvements.
Despite these limitations, there are many possible applications, as the transmission model can be combined with field measurements for risk prioritization, or with simulated pedestrian trajectories. In this way, for example, we can quantify in advance the influence of architectural choices on the risk of transmission in a building, or organize the circulation of a crowd during an epidemic.
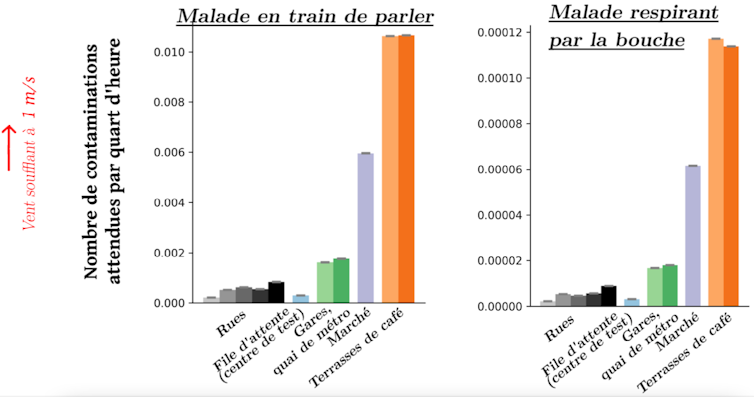
Simon Mendez, CNRS Research Fellow, Mathematics and Modeling Laboratory, University of Montpellier and Alexandre Nicolas, CNRS research fellow; physicist, Université Claude Bernard Lyon 1
This article is republished from The Conversation under a Creative Commons license. Read theoriginal article.