From Facebook to plant development: when networks get involved
Telephone network, social network, road network, professional network... The term "network" has become part of everyday language. A computer network made up of interconnected computers, or a subway network whose stations are connected by lines, are two intuitive representations.
Jérémy Lavarenne, Institute of Research for Development (IRD)
Quinn Dombrowski/Flickr, CC BY-SA
Visit graph theoryNetwork theory, transposed to the real world through network theory, teaches us that networks have intrinsic mathematical properties. These make it possible to study them formally, and thus to better understand and even anticipate the functioning of the systems they describe.
What is a network?
The Wiktionary defines a network as "a set of objects or persons connected or maintained in liaison", but also as "the set of relationships thus established". There are several ways of representing a network, the most common and intuitive of which is in the form of a mathematical object called a graph. This is defined as a set of nodes and edges interconnecting these nodes. It can include an extra layer of information, such as the addition of a weight value to nodes to represent the relative importance of an object, or the directionality of edges to distinguish the direction of the relationship between two objects. For example, on the Twitter microblogging network, the connection between a follower and the account being followed is not necessarily reciprocal: the interaction is directed, and could be represented by an arrow starting from the follower and pointing towards the account being followed.
Internet, ecosystems, the human brain, economic organizations... Graphs can be used to represent - or "model" - and analyze a wide variety of complex systems. This makes these approaches all the more interesting, as complex systems are defined by a behavior that is difficult to understand and anticipate on the basis of individual knowledge of their components alone.
Network analysis: a broad spectrum of applications
Coupled with ever-increasing computing performance, the contributions of graph theory and advances in algorithmics provide us with a set of tools that enable us to extract information from large-scale networks. Indeed, it's not uncommon to encounter graphs made up of thousands of nodes and tens of thousands of edges, whose information may seem difficult to grasp at first glance.
A familiar and amusing example of the benefits of network analysis comes from the social sciences. Determined by Wayne W. Zachary between 1970 and 1972, Zachary's Karate Club is a graph documenting 78 affinity links between 34 members of a martial arts club. Interested in the role of information and sentimental flows in the splitting of small groups, the author applied an algorithm that grouped together the members with the greatest affinity to hypothesize the formation of sub-groups that might result from conflict. Amusingly, such an event occurred during the course of the study, causing an actual split in the group, identical to the one predicted by the affinity network analysis, and validating Zachary's model by 97%.
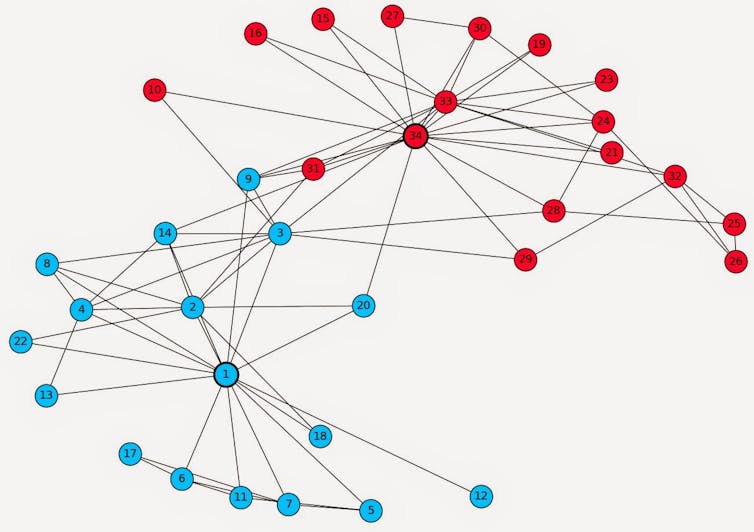
Historical DataNinjas blog
Another example, borrowed from the field of ecology and brought to life in 2016 by Mauro Martino on the basis of research by Guo and colleagues, presents us with a network made up of different plant species (nodes) sharing at least one species of symbiotic ant (edges). Here, analysis of the network in the context of a simulation of the gradual, random disappearance of certain ant species as a result of climate change enables us to estimate the network's resilience, i.e. its ability to withstand environmental variations while remaining capable of performing its functions. This approach thus provides an additional indicator to others already in existence to describe the fragility of ecosystems in the face of climate change.
Cereal root formation
Climate change is also set to have a major impact on agricultural activities and yields, with changes in rainfall patterns leading to more severe weather episodes. To compensate for these fluctuations, agronomists and biologists agree on the importance of working on the root system, the "hidden half" of plants. This is in the front line when the soil is short of water (drought) or contaminated by excessive levels of sodium chloride (salinization). Cereals, and in particular the Poaceae family which includes rice, corn and wheat, are the direct or indirect source of almost half of mankind's food calories. To adapt plants to the consequences of global climate change, it is therefore strategic to understand the genetic determinants of root system architecture. These may control, for example, the number or diameter of roots, or the angle or depth of rooting. On the other hand, it is important to understand the role of this architecture in making plants tolerant to these stresses.
In this context, my thesis work is focused on understanding the early stages of root development in rice. At first glance, there's no network in sight! However, one aspect of network science is closely linked to biology: the study of gene regulatory networks.
During a developmental process such as rootorganogenesis, specific genes are translated into proteins. Some of these proteins are involved in the regulation of other genes. One way of studying a biological process is to identify the links between, on the one hand, genes coding for regulators and, on the other, the genes whose expression they regulate, in order to begin modeling a gene regulatory network.
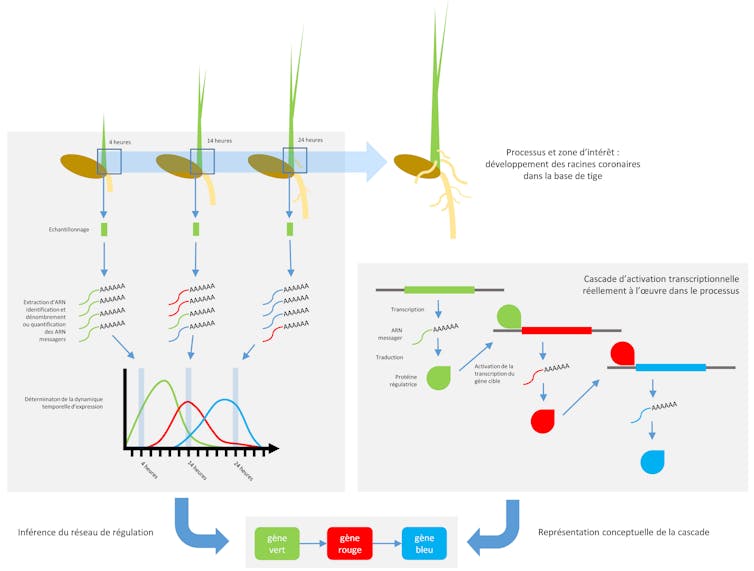
This is what we've done to study and discover new genetic determinants involved in the formation of coronal roots, a class of roots that emerge in a crown from the stem internodes as the plant develops, and in cereals make up the major part of the root system.
These coronal roots form the major part of the root system in cereals. To this end, we sampled the organ concerned at regular intervals throughout the early stages of root formation in rice. Thanks to recent advances in transcriptomics techniques, we were able to identify variations in gene expression levels during the development of a coronal root in rice. From a subset of genes showing strong variations in expression levels, we have, thanks to an algorithm that analyzes similarities and temporal shifts between gene expression profiles over time, predicted which gene regulates which other. On this basis, we built a model of a gene regulatory network that could explain these expression variations.
What applications?
From a fundamental point of view, the main interest of this network analysis is to identify new links between genes already described for their involvement in root development, but also with genes whose role in this process was previously unknown. Also, analysis of this network using clustering methods (as presented in the Zachary example) enables us to identify groups of genes that are more strongly connected to each other than to the rest of the network. Such groups, known as modules, are likely to play a role in a given biological function. Finally, by identifying the most highly connected nodes in the network, we can pinpoint genes whose role is crucial to the process as a whole.
In this respect, these so-called "systems biology" approaches, which take into account the globality of a process rather than focusing on certain of its elements, are interesting from a fundamental point of view for formulating new hypotheses on how it works. From an applied point of view, they can help detect new target genes for varietal selection, for example in our case to modulate root architecture and obtain plants that are more tolerant to stresses such as drought. Finally, whether in fundamental or applied research, the predictive capacities of systems approaches could reduce the time needed to create new plant varieties.
Recent advances in sequencing technologies (such as RNAseq, for "RNA sequencing") and methods enabling the modelling and analysis of gene regulatory networks open up the latter for use on an ever wider range of crop species. This leads us to believe that varietal selection activities, hitherto based on the selection of gene variants without consideration of their role in gene regulation, will be enriched by systems biology in the years to come. Breeders could thus integrate "regulatory network engineering" functions. Varietal crosses and biotechnological tools would then have a role to play in adding or rewiring network nodes controlling specific aspects of plant growth or stress response.
To find out more about network science, we recommend reading the book "Network science" by Albert-László Barabási, freely available on the author's website. We would like to thank Antony Champion (IRD) for proofreading this text..
Jérémy LavarenneAgricultural engineer, doctoral student CIFRE University of Montpellier-Biogemma, Institute of Research for Development (IRD)
Visit original version of this article was published on The Conversation.