Tardigrades in quantum country?
Biology, including "molecular" biology, has traditionally been the closed field of the comfortable classical sciences, while physics and chemistry have been infiltrated by the bewitching waves of quantum physics. But recently, remarkable advances on the biotechnical front have made it possible to explore the quantum aspect of life.
Simon Galas, University of Montpellier and Michel Cassé, French Atomic Energy and Alternative Energies Commission (CEA)
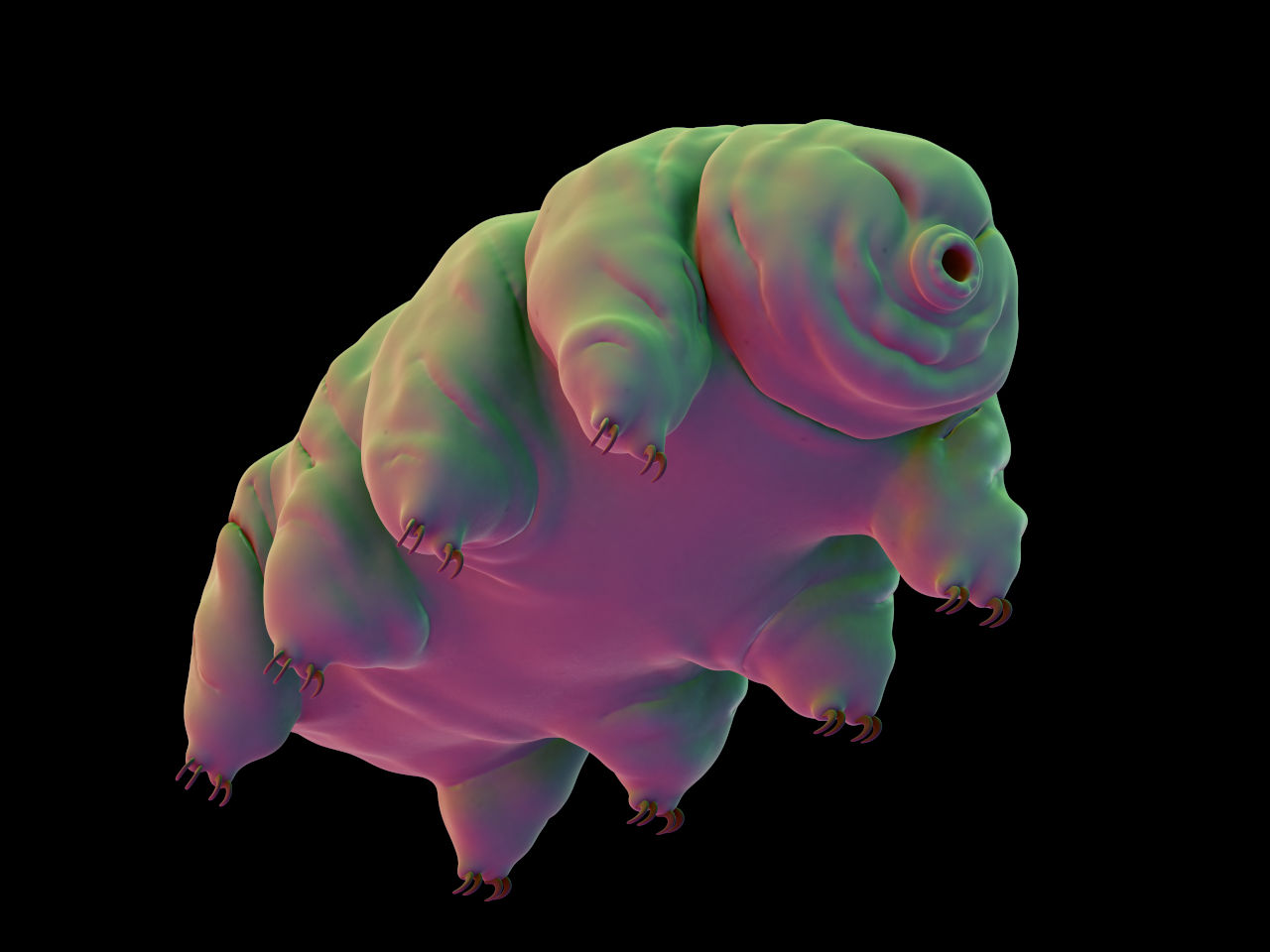
SciePro/Shutterstock
The term "quantum biology" covers the application of concepts from the physics of atoms and their components to the biological science of cells and whole organisms. Its main areas of application are enzyme-catalyzed reactions, photosynthesis, ion channels, olfaction, the navigation of migratory birds and the tunneling of protons into DNA.
Is quantum mechanics, random and capricious, a sign of what gives life its impetus and charm? Does the intertwining, weaving and embroidery of the biological and the elementary quantum promise to reveal the rules of fundamental life in the sense of fundamental science? Does life require quantum mechanics? Yes, of course: we're made of atoms, and our physical-chemical machinery is molecular. But in a more subtle way, can we hope to shed light on phenomena that today challenge us to understand them? In other words, does quantum mechanics play a fundamental role in biology? Does it have a physiological impact? And if so, what future biomimetic applications does it herald?
It's against this backdrop of open-ended technology that we see the emergence of four-legged animals with unusual stamina, known as tardigrades, because they walk slowly. These animals were the subject of a recent experiment that made headlines and caused a stir in the quantum and biological communities, while leaving some researchers sceptical.
The embrace of tardigrade and qubit
Researchers in Singapore have sought to associate a cryogenically frozen tardigrade with a qubit (an essential component of superconducting circuits).
Electrons and photons behave in a non-conformist way: we can't say exactly where they are, in what direction or how fast they're moving. However, a mathematical tool called a wave function enables us to calculate where the electron or photon probably is, and where it's probably headed. Properly isolated and left to its own devices, a pure quantum state described by a coherent wave function evolves predictively, according to a mathematical prescription well established by Schrödinger. But when measurement occurs (in the broad sense of interaction with a macroscopic object), the state changes abruptly. Quiet evolution is interrupted and replaced by a roulette destiny. Schrödinger's perfectly deterministic equation gives way to Born's rule, which predicts the relative probabilities of various measurement results, introducing an element of indeterminism and uncertainty in the process. This conceptual catastrophe marks the transition between the quantum and classical realms.
Quantum entanglement, on the other hand, refers to a relationship of dependence between particles that have interacted, however far apart they may be. (Note: Schrödinger saw entanglement as the hallmark of quantum physics, while Einstein cursed it as "spooky action at a distance").
But back to our tardigrades. In their experiment, Reiner Damke and his colleagues at Nanyang Technological University in Singapore integrated the tardigrade into a circuit consisting of two superconducting qubits.
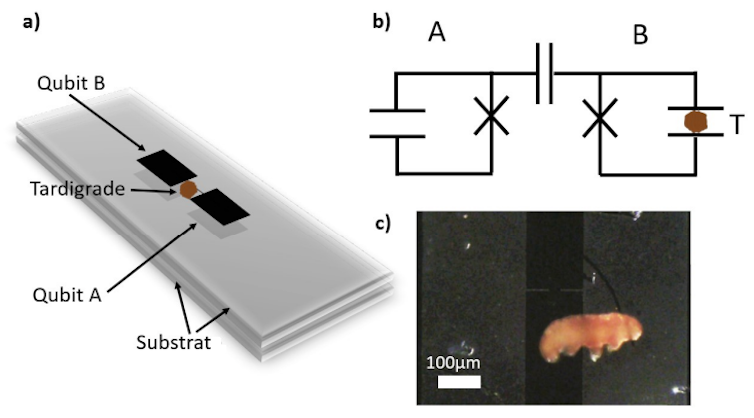
K.S. Lee et al./arXiv
Next, they gradually lowered the pressure and temperature of the enclosure to create the most perfect laboratory vacuum possible, in order to reduce external influences on the qubits and the small animal. Finally, they measured the frequencies at which the tardigrade-qubit combination vibrated. The result? The protagonists were in a state of quantum superposition: their properties were no longer independent (in other words, information from one was needed to describe the other, and vice versa).
Then, as the enclosure returned to more natural temperature and pressure levels, the tardigrade was warmed up and brought out of hibernation to resume its life. In other words, the little beast entered the collectivist, sharing and impersonal quantum world, then returned to its solitary, well-differentiated condition at the end of the operation, without seeming to have suffered from this strange journey.
Accolade, really?
Faced with this result, metaphysical questions invade the mind. What does it mean for a living being to be "intertwined"? And marrying a metal object? It opens the door to all kinds of fantasies. To avoid any flights of fancy, it's a good idea to put the situation into perspective.
First of all, the temperature and pressure conditions under which the experiment was carried out are extremely coercive. Such conditions are unlikely to occur frequently in nature!
As for the tardigrade, it was cryogenically frozen, which changes everything! Ice acts like a dielectric, modifying the resonant frequency of the qubit on which it is placed. It was therefore logical to observe a change in vibratory frequency, whether the ice was a frozen tardigrade or a simple ice cube, and any other material modifying the electric field would have led to the same result. To consider this as quantum entanglement would be equivalent to saying that a qubit, under the usual conditions, is entangled with the silicon chip that supports it.
In short, we're still a long way from real quantum entanglement involving living organisms. Malicious tongues claim that this feat is more the fault of the tardigrade than of the researchers...
Simon Galas, Professor of Genetics and Molecular Biology of Aging, IBMM CNRS UMR 5247 - Faculty of Pharmacy, University of Montpellier and Michel Cassé, Astrophysicist and writer, French Atomic Energy and Alternative Energies Commission (CEA)
This article is republished from The Conversation under a Creative Commons license. Read theoriginal article.